Mastering Fluid-Structure Interaction (FSI) Simulation in Abaqus: A Comprehensive Guide
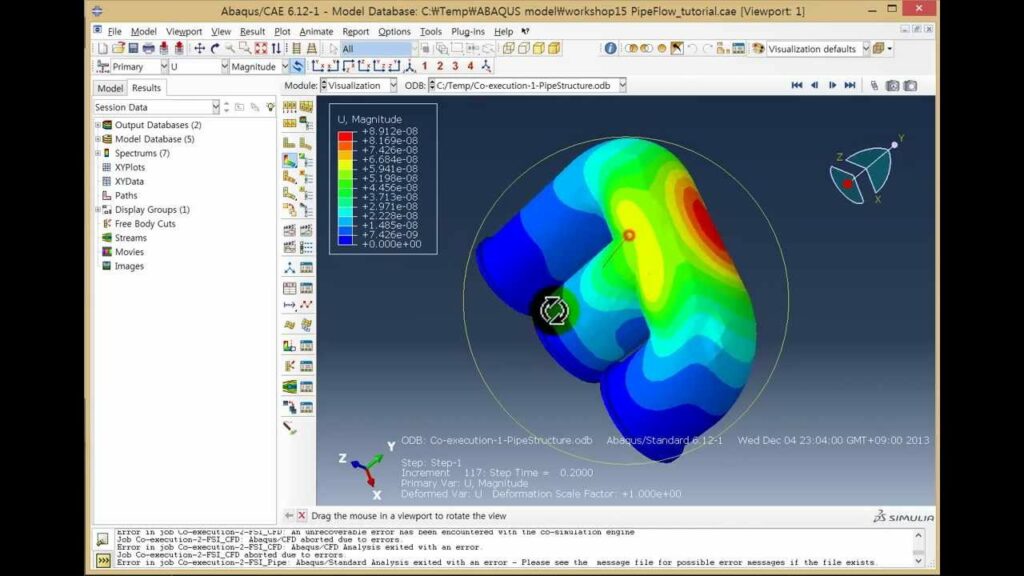
Introduction: Fluid-Structure Interaction (FSI) is a complex engineering phenomenon that occurs when the behavior of a fluid affects the deformation and response of adjacent solid structures, and vice versa. FSI simulations are critical for understanding and predicting the performance of systems such as aircraft wings, wind turbines, and cardiovascular devices. Abaqus, developed by Dassault Systèmes, is a powerful finite element analysis software suite that offers advanced capabilities for simulating FSI problems. In this comprehensive guide, we will explore the intricacies of simulating fluid-structure interaction in Abaqus, covering everything from problem setup and modeling techniques to solution strategies and result interpretation.
Section 1: Introduction to Fluid-Structure Interaction 1.1 Understanding Fluid-Structure Interaction: Fluid-Structure Interaction (FSI) refers to the interaction between a fluid and a solid structure, where the motion and deformation of one component influence the behavior of the other. FSI phenomena are prevalent in engineering applications such as aerodynamics, hydrodynamics, and biomechanics, where the coupling between fluid flow and structural response plays a significant role in system performance and stability.
1.2 Importance of FSI Simulation: FSI simulation enables engineers to predict the dynamic behavior of fluid-structure systems, assess their structural integrity, and optimize their design for performance and reliability. By simulating the interaction between fluids and structures, engineers can analyze phenomena such as flow-induced vibrations, fluid-structure resonance, and hydrodynamic loading, leading to improved designs and reduced development costs.
Section 2: Setting Up FSI Simulations in Abaqus 2.1 Overview of Abaqus: Abaqus is a finite element analysis software suite that offers a wide range of simulation capabilities, including structural analysis, heat transfer, and multiphysics simulations. Abaqus provides specialized features and modules for simulating fluid-structure interaction problems, allowing engineers to model complex interactions between fluids and structures accurately.
2.2 Preparing the Simulation Environment: Before setting up an FSI simulation in Abaqus, engineers need to define the simulation environment, including the fluid domain, solid structure, and boundary conditions. Engineers use Abaqus/CAE, the graphical user interface for Abaqus, to create the simulation model, define material properties, and specify loading and boundary conditions for both the fluid and solid domains.
Section 3: Modeling Fluid Domains in Abaqus 3.1 Fluid Meshing and Boundary Conditions: Abaqus provides tools for generating high-quality meshes for fluid domains, including tetrahedral, hexahedral, and hybrid meshing techniques. Engineers can refine the mesh near solid-fluid interfaces and boundary layers to capture flow gradients and turbulence effects accurately. Additionally, engineers specify boundary conditions such as velocity inlets, pressure outlets, and wall conditions to define fluid flow behavior within the domain.
3.2 Fluid Material Properties and Properties: In Abaqus, engineers define fluid material properties such as density, viscosity, and thermal conductivity to characterize the behavior of the fluid medium. Engineers can model Newtonian or non-Newtonian fluids, as well as multiphase flows and compressible fluids, depending on the specific application requirements.
Section 4: Modeling Solid Structures in Abaqus 4.1 Solid Meshing and Boundary Conditions: For solid structures, engineers use Abaqus to generate finite element meshes that accurately capture the geometry and material properties of the structure. Engineers refine the mesh in regions of interest, such as areas of high stress or deformation, to ensure accurate simulation results. Additionally, engineers apply boundary conditions such as fixed constraints, applied loads, and contact interactions to represent the structural behavior under external forces and constraints.
4.2 Solid Material Properties and Constitutive Models: In Abaqus, engineers define material properties and constitutive models to characterize the mechanical behavior of solid materials under loading conditions. Engineers can choose from a wide range of material models, including linear elastic, nonlinear elastic, plasticity, and viscoelasticity, to simulate the response of solid structures under various loading scenarios.
Section 5: Coupling Fluid and Solid Domains 5.1 Fluid-Structure Interaction Coupling: In Abaqus, engineers establish coupling between fluid and solid domains using specialized coupling algorithms and techniques. Abaqus offers several methods for solving fluid-structure interaction problems, including one-way coupling, two-way coupling, and partitioned coupling approaches. Engineers select the appropriate coupling method based on the physics of the problem, computational efficiency, and numerical stability requirements.
5.2 Interface Definition and Communication: To facilitate communication between fluid and solid domains, engineers define interfaces and interaction surfaces at the solid-fluid interface. Abaqus provides tools for specifying contact conditions, interface elements, and coupling constraints to ensure continuity of displacements, velocities, and pressures across the interface. Engineers may also define coupling parameters such as time step sizes, convergence criteria, and interpolation schemes to control the FSI solution process.
Section 6: Solving and Post-Processing FSI Simulations 6.1 Solution Strategies and Algorithms: In Abaqus, engineers employ advanced solution strategies and algorithms to solve FSI problems efficiently and accurately. Abaqus utilizes implicit and explicit solution techniques, coupled with iterative solvers and time integration schemes, to simulate transient fluid-structure interactions with high fidelity and numerical stability. Engineers may adjust solution settings such as time step sizes, convergence tolerances, and solver options to achieve optimal performance and accuracy.
6.2 Post-Processing and Result Analysis: Once the simulation is complete, engineers use Abaqus to post-process and analyze the simulation results. Abaqus provides powerful visualization tools for plotting contours, vectors, and animations of fluid flow fields, structural deformations, and coupling forces. Engineers interpret simulation data, extract key performance metrics, and evaluate the structural integrity, fluid behavior, and overall system response to validate the simulation results and inform design decisions.
Section 7: Real-World Applications and Case Studies 7.1 Aerospace and Aeronautics: In the aerospace industry, Abaqus is used to simulate fluid-structure interaction phenomena in aircraft wings, fuselages, and control surfaces. Engineers model aerodynamic loads, structural deformations, and aeroelastic effects to assess the stability, performance, and safety of aircraft structures under various flight conditions.
7.2 Biomedical Engineering: In biomedical engineering, Abaqus enables researchers to simulate fluid-structure interactions in cardiovascular devices such as heart valves, stents, and blood vessels. Engineers model blood flow, tissue deformation, and fluid-structure interactions to study the biomechanical behavior of cardiovascular implants and optimize their design for durability and biocompatibility.
Section 8: Best Practices and Optimization Strategies 8.1 Model Simplification and Mesh Refinement: To improve computational efficiency and solution accuracy, engineers should simplify the simulation model and refine the mesh in critical regions. Engineers use domain decomposition techniques, adaptive meshing algorithms, and mesh convergence studies to achieve the optimal balance between simulation accuracy and computational cost.
8.2 Validation and Verification: Before applying FSI simulations to real-world engineering problems, engineers should validate and verify the simulation models against experimental data or analytical solutions. Engineers perform benchmark tests, sensitivity analyses, and model validation studies to ensure that the simulation results are reliable, robust, and consistent with physical observations.
Section 9: Future Trends and Developments 9.1 High-Performance Computing (HPC): As computing power continues to increase, future developments in FSI simulation may focus on leveraging high-performance computing (HPC) resources to accelerate simulation workflows and enable larger-scale simulations. Engineers may exploit parallel processing, distributed computing, and cloud computing technologies to tackle complex FSI problems with greater efficiency and scalability.
9.2 Multiphysics Coupling and Optimization: Future versions of Abaqus may enhance multiphysics coupling capabilities and optimization algorithms to enable more seamless integration of fluid-structure interaction with other physical phenomena such as heat transfer, acoustics, and electromagnetic fields. Engineers may explore multiphysics simulation approaches to capture the interplay between different physical processes and optimize system performance across multiple domains.
Conclusion: Fluid-Structure Interaction (FSI) simulation in Abaqus offers engineers a powerful and versatile tool for analyzing complex interactions between fluids and structures in engineering systems. By mastering the techniques and best practices outlined in this guide, engineers can leverage Abaqus’s advanced capabilities to model, simulate, and optimize fluid-structure interaction problems with confidence and efficiency. With its robust solution algorithms, intuitive user interface, and extensive post-processing tools, Abaqus continues to be a preferred choice for engineers seeking to address challenging FSI problems and advance the state-of-the-art in fluid-structure interaction simulation across diverse industries and applications.